National Aeronautics and Space Administration
Goddard space flight center, imagine the universe, astronomer's toolbox.
- Cosmic Objects
- Big Questions
- Featured Science
- Observatories
- Scientist Profiles
- You Be the Astrophysicist
- The Cosmic Distance Scale
- Lesson Plans
- Ask an Astrophysicist
- Other Resources
- News #include virtual="/news/newsNav.html"

Electromagnetic Spectrum: Quiz

- Project Leader: Dr. Barbara Mattson
- Web Curator: J.D. Myers
- Responsible NASA Official : Dr. Andy Ptak
- Privacy Policy & Important Notices
- Page Last Updated: 05-Nov-2013
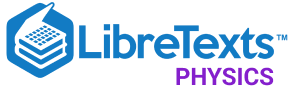
- school Campus Bookshelves
- menu_book Bookshelves
- perm_media Learning Objects
- login Login
- how_to_reg Request Instructor Account
- hub Instructor Commons
- Download Page (PDF)
- Download Full Book (PDF)
- Periodic Table
- Physics Constants
- Scientific Calculator
- Reference & Cite
- Tools expand_more
- Readability
selected template will load here
This action is not available.
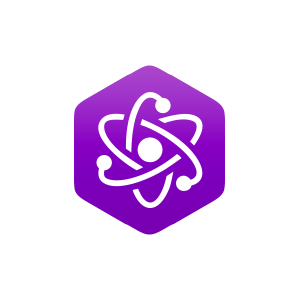
16.2: Maxwell’s Equations and Electromagnetic Waves
- Last updated
- Save as PDF
- Page ID 4449
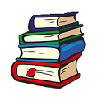
Learning Objectives
By the end of this section, you will be able to:
- Explain Maxwell’s correction of Ampère’s law by including the displacement current
- State and apply Maxwell’s equations in integral form
- Describe how the symmetry between changing electric and changing magnetic fields explains Maxwell’s prediction of electromagnetic waves
- Describe how Hertz confirmed Maxwell’s prediction of electromagnetic waves
James Clerk Maxwell (1831–1879) was one of the major contributors to physics in the nineteenth century (Figure \(\PageIndex{1}\)). Although he died young, he made major contributions to the development of the kinetic theory of gases, to the understanding of color vision, and to the nature of Saturn’s rings. He is probably best known for having combined existing knowledge of the laws of electricity and of magnetism with insights of his own into a complete overarching electromagnetic theory, represented by Maxwell’s equations .
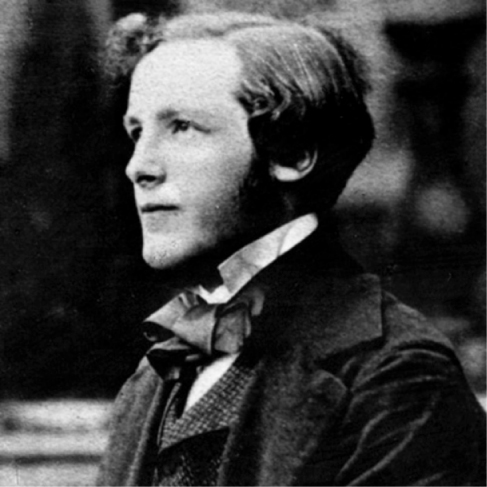
Maxwell’s Correction to the Laws of Electricity and Magnetism
The four basic laws of electricity and magnetism had been discovered experimentally through the work of physicists such as Oersted, Coulomb, Gauss, and Faraday. Maxwell discovered logical inconsistencies in these earlier results and identified the incompleteness of Ampère’s law as their cause.
Recall that according to Ampère’s law, the integral of the magnetic field around a closed loop C is proportional to the current I passing through any surface whose boundary is loop C itself:
\[\oint \vec{B} \cdot d\vec{s} = \mu_0 I. \label{Eq1}\]
There are infinitely many surfaces that can be attached to any loop, and Ampère’s law stated in Equation \ref{Eq1} is independent of the choice of surface.
Consider the set-up in Figure \(\PageIndex{2}\). A source of emf is abruptly connected across a parallel-plate capacitor so that a time-dependent current I develops in the wire. Suppose we apply Ampère’s law to loop C shown at a time before the capacitor is fully charged, so that \( I \neq 0\). Surface \(S_1\) gives a nonzero value for the enclosed current I , whereas surface \(S_2\) gives zero for the enclosed current because no current passes through it:
\[\underbrace{\oint_C \vec{B} \cdot d\vec{s} = \mu_0 I}_{\text{if surface } S_1 \text{is used}}\]
\[\underbrace{ \, =0 }_{\text{if surface } S_2 \text{is used}}\]
Clearly, Ampère’s law in its usual form does not work here. This may not be surprising, because Ampère’s law as applied in earlier chapters required a steady current, whereas the current in this experiment is changing with time and is not steady at all.
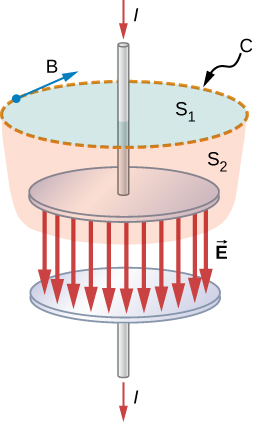
How can Ampère’s law be modified so that it works in all situations? Maxwell suggested including an additional contribution, called the displacement current \(I_d\), to the real current I ,
\[\boxed{\oint_S \vec{B} \cdot d\vec{s} = \mu_0 (I + I_d)} \label{EQ4}\]
where the displacement current is defined to be
\[\boxed{I_d = \epsilon_0 \dfrac{d\Phi_E}{dt}.} \label{EQ5}\]
Here \(\epsilon_0\) is the permittivity of free space and \(\Phi_E\) is the electric flux , defined as
\[\Phi_E = \iint_{Surface \, S} \vec{E} \cdot d\vec{A}.\]
The displacement current is analogous to a real current in Ampère’s law, entering into Ampère’s law in the same way. It is produced, however, by a changing electric field. It accounts for a changing electric field producing a magnetic field, just as a real current does, but the displacement current can produce a magnetic field even where no real current is present. When this extra term is included, the modified Ampère’s law equation becomes
\[\oint_C \vec{B} \cdot d\vec{s} = \mu_0 I + \epsilon_0 \mu_0 \dfrac{d\Phi_E}{dt}\]
and is independent of the surface S through which the current I is measured.
We can now examine this modified version of Ampère’s law to confirm that it holds independent of whether the surface \(S_1\) or the surface \(S_2\) in Figure \(\PageIndex{2}\) is chosen. The electric field \(\vec{E}\) corresponding to the flux \(\Phi_E\) in Equation \ref{EQ5} is between the capacitor plates. Therefore, the \(\vec{E}\) field and the displacement current through the surface \(S_1\) are both zero, and Equation \ref{EQ4} takes the form
\[\oint_C \vec{B} \cdot d\vec{s} = \mu_0 I. \label{16.5}\]
We must now show that for surface \(S_2\), through which no actual current flows, the displacement current leads to the same value \(\mu_0 I\) for the right side of the Ampère’s law equation. For surface \(S_2\) the equation becomes
\[\oint_C \vec{B} \cdot d\vec{s} = \mu_0 \dfrac{d}{dt} \left[ \epsilon_0 \iint_{Surface \, S_2} \vec{E} \cdot d\vec{A} \right].\]
Gauss’s law for electric charge requires a closed surface and cannot ordinarily be applied to a surface like \(S_1\) alone or \(S_2\) alone. But the two surfaces \(S_1\) and \(S_2\) form a closed surface in Figure \(\PageIndex{2}\) and can be used in Gauss’s law. Because the electric field is zero on \(S_1\), the flux contribution through \(S_1\) is zero. This gives us
\[ \begin{align} \oint_{Surface \, S_1 + S_2} \vec{E} \cdot d\vec{A} &= \iint_{Surface \, S_1} \vec{E} \cdot d\vec{A} + \iint_{Surface \, S_2} \vec{E} \cdot d\vec{A} \\[4pt] &= 0 + \iint_{Surface \, S_2} \vec{E} \cdot d\vec{A} \\[4pt] &= \iint_{Surface \, S_2} \vec{E} \cdot d\vec{A}. \end{align}\]
Therefore, we can replace the integral over \(S_2\) in Equation \ref{16.5} with the closed Gaussian surface \(S_1 + S_2\) and apply Gauss’s law to obtain
\[\oint_{S_1} \vec{B} \cdot d\vec{s} = \mu_0 \dfrac{dQ_{in}}{dt} = \mu_0 I.\]
Thus, the modified Ampère’s law equation is the same using surface \(S_2\), where the right-hand side results from the displacement current, as it is for the surface \(S_1\), where the contribution comes from the actual flow of electric charge.
Displacement current in a charging capacitor
A parallel-plate capacitor with capacitance C whose plates have area A and separation distance d is connected to a resistor R and a battery of voltage V . The current starts to flow at \(t = 0\).
- Find the displacement current between the capacitor plates at time t .
- From the properties of the capacitor, find the corresponding real current \(I = \dfrac{dQ}{dt}\), and compare the answer to the expected current in the wires of the corresponding RC circuit.
We can use the equations from the analysis of an RC circuit ( Alternating-Current Circuits ) plus Maxwell’s version of Ampère’s law.
- The voltage between the plates at time t is given by \[V_C = \dfrac{1}{C} Q(t) = V_0 \left(1 - e^{-t/RC}\right). \nonumber\] Let the z -axis point from the positive plate to the negative plate. Then the z -component of the electric field between the plates as a function of time t is \[E_z (t) = \dfrac{V_0}{d}\left(1 - e^{-t/RC}\right). \nonumber\] Therefore, the z-component of the displacement current \(I_d\) between the plates is \[I_d (t) = \epsilon_0 A\dfrac{\partial E_z (t)}{\partial t} = \epsilon_0 A \dfrac{V_0}{d} \times \dfrac{1}{RC} e^{-t/RC} = \dfrac{V_0}{R} e^{-t/RC}, \nonumber\] where we have used \(C = \epsilon_0 \dfrac{A}{d}\) for the capacitance.
- From the expression for \(V_C\) the charge on the capacitor is \[Q(t) = CV_C = CV_0 \left(1 - e^{-t/RC}\right). \nonumber\] The current into the capacitor after the circuit is closed, is therefore \[I = \dfrac{dQ}{dt} = \dfrac{V_0}{R}e^{-t/RC}. \nonumber\] This current is the same as \(I_d\) found in (a).
Maxwell’s Equations
With the correction for the displacement current, Maxwell’s equations take the form
\[ \begin{align} \oint \vec{E} \cdot d\vec{A} &= \dfrac{Q_{in}}{\epsilon_0} \, (\text{Gauss's law}) \label{eq1} \\[4pt] \oint \vec{B} \cdot d\vec{A} &= 0 \, (\text{Gauss's law for magnetism}) \label{eq2} \\[4pt] \oint \vec{E} \cdot d\vec{s} &= - \dfrac{d\Phi_m}{dt} \, (\text{Faraday's law}) \label{eq3} \\[4pt] \oint \vec{B} \cdot d\vec{s} &= \mu_0 I + \epsilon_0I + \epsilon_0 \mu_0 \dfrac{d\Phi_E}{dt} (\text{Ampere-Maxwell law}). \label{eq4} \end{align}\]
Once the fields have been calculated using these four equations, the Lorentz force equation
\[\vec{F} = q\vec{E} + q\vec{v} \times \vec{B}\]
gives the force that the fields exert on a particle with charge q moving with velocity \(\vec{v}\). The Lorentz force equation combines the force of the electric field and of the magnetic field on the moving charge. The magnetic and electric forces have been examined in earlier modules. These four Maxwell’s equations are, respectively:
Maxwell's Equations
1. Gauss’s law
The electric flux through any closed surface is equal to the electric charge \(Q_{in}\) enclosed by the surface. Gauss’s law (Equation \ref{eq1}) describes the relation between an electric charge and the electric field it produces. This is often pictured in terms of electric field lines originating from positive charges and terminating on negative charges, and indicating the direction of the electric field at each point in space.
2. Gauss’s law for magnetism
The magnetic field flux through any closed surface is zero (Equation \ref{eq2}). This is equivalent to the statement that magnetic field lines are continuous, having no beginning or end. Any magnetic field line entering the region enclosed by the surface must also leave it. No magnetic monopoles, where magnetic field lines would terminate, are known to exist (see section on Magnetic Fields and Lines ).
3. Faraday’s law
A changing magnetic field induces an electromotive force (emf) and, hence, an electric field. The direction of the emf opposes the change. Equation \ref{eq3} is Faraday’s law of induction and includes Lenz’s law. The electric field from a changing magnetic field has field lines that form closed loops, without any beginning or end.
4. Ampère-Maxwell law
Magnetic fields are generated by moving charges or by changing electric fields. This fourth of Maxwell’s equations, Equation \ref{eq4}, encompasses Ampère’s law and adds another source of magnetic fields, namely changing electric fields.
Maxwell’s equations and the Lorentz force law together encompass all the laws of electricity and magnetism. The symmetry that Maxwell introduced into his mathematical framework may not be immediately apparent. Faraday’s law describes how changing magnetic fields produce electric fields. The displacement current introduced by Maxwell results instead from a changing electric field and accounts for a changing electric field producing a magnetic field. The equations for the effects of both changing electric fields and changing magnetic fields differ in form only where the absence of magnetic monopoles leads to missing terms. This symmetry between the effects of changing magnetic and electric fields is essential in explaining the nature of electromagnetic waves.
Later application of Einstein’s theory of relativity to Maxwell’s complete and symmetric theory showed that electric and magnetic forces are not separate but are different manifestations of the same thing—the electromagnetic force. The electromagnetic force and weak nuclear force are similarly unified as the electroweak force. This unification of forces has been one motivation for attempts to unify all of the four basic forces in nature—the gravitational, electrical, strong, and weak nuclear forces (see Particle Physics and Cosmology ).
The Mechanism of Electromagnetic Wave Propagation
To see how the symmetry introduced by Maxwell accounts for the existence of combined electric and magnetic waves that propagate through space, imagine a time-varying magnetic field \(\vec{B}_0(t)\) produced by the high-frequency alternating current seen in Figure \(\PageIndex{3}\). We represent \(\vec{B}_0(t)\) in the diagram by one of its field lines. From Faraday’s law, the changing magnetic field through a surface induces a time-varying electric field \(\vec{E}_0(t)\) at the boundary of that surface. The displacement current source for the electric field, like the Faraday’s law source for the magnetic field, produces only closed loops of field lines, because of the mathematical symmetry involved in the equations for the induced electric and induced magnetic fields. A field line representation of \(\vec{E}_0(t)\) is shown. In turn, the changing electric field \(\vec{E}_0(t)\) creates a magnetic field \(\vec{B}_1(t)\) according to the modified Ampère’s law. This changing field induces \(\vec{E}_1(t)\) which induces \(\vec{B}_2(t)\) and so on. We then have a self-continuing process that leads to the creation of time-varying electric and magnetic fields in regions farther and farther away from O . This process may be visualized as the propagation of an electromagnetic wave through space.
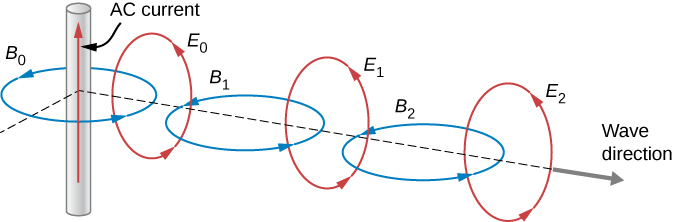
In the next section, we show in more precise mathematical terms how Maxwell’s equations lead to the prediction of electromagnetic waves that can travel through space without a material medium, implying a speed of electromagnetic waves equal to the speed of light.
Prior to Maxwell’s work, experiments had already indicated that light was a wave phenomenon, although the nature of the waves was yet unknown. In 1801, Thomas Young (1773–1829) showed that when a light beam was separated by two narrow slits and then recombined, a pattern made up of bright and dark fringes was formed on a screen. Young explained this behavior by assuming that light was composed of waves that added constructively at some points and destructively at others (see Interference ). Subsequently, Jean Foucault (1819–1868), with measurements of the speed of light in various media, and Augustin Fresnel (1788–1827), with detailed experiments involving interference and diffraction of light, provided further conclusive evidence that light was a wave. So, light was known to be a wave, and Maxwell had predicted the existence of electromagnetic waves that traveled at the speed of light. The conclusion seemed inescapable: Light must be a form of electromagnetic radiation. But Maxwell’s theory showed that other wavelengths and frequencies than those of light were possible for electromagnetic waves. He showed that electromagnetic radiation with the same fundamental properties as visible light should exist at any frequency. It remained for others to test, and confirm, this prediction.
Exercise \(\PageIndex{1}\)
When the emf across a capacitor is turned on and the capacitor is allowed to charge, when does the magnetic field induced by the displacement current have the greatest magnitude?
It is greatest immediately after the current is switched on. The displacement current and the magnetic field from it are proportional to the rate of change of electric field between the plates, which is greatest when the plates first begin to charge.
Hertz’s Observations
The German physicist Heinrich Hertz (1857–1894) was the first to generate and detect certain types of electromagnetic waves in the laboratory. Starting in 1887, he performed a series of experiments that not only confirmed the existence of electromagnetic waves but also verified that they travel at the speed of light.
Hertz used an alternating-current RLC (resistor-inductor-capacitor) circuit that resonates at a known frequency \(f_0 = \dfrac{1}{2\pi \sqrt{LC}}\) and connected it to a loop of wire, as shown in Figure \(\PageIndex{4}\). High voltages induced across the gap in the loop produced sparks that were visible evidence of the current in the circuit and helped generate electromagnetic waves.
Across the laboratory, Hertz placed another loop attached to another RLC circuit, which could be tuned (as the dial on a radio) to the same resonant frequency as the first and could thus be made to receive electromagnetic waves. This loop also had a gap across which sparks were generated, giving solid evidence that electromagnetic waves had been received.
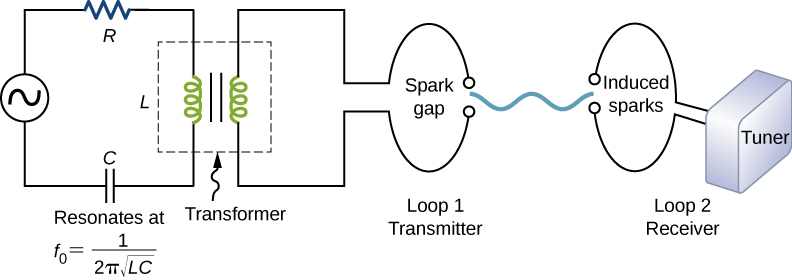
Hertz also studied the reflection, refraction, and interference patterns of the electromagnetic waves he generated, confirming their wave character. He was able to determine the wavelengths from the interference patterns, and knowing their frequencies, he could calculate the propagation speed using the equation \(v = f\lambda\), where v is the speed of a wave, f is its frequency, and \(\lambda\) is its wavelength. Hertz was thus able to prove that electromagnetic waves travel at the speed of light. The SI unit for frequency, the hertz \((1 \, Hz = 1 \, cycle/second)\), is named in his honor.
Exercise \(\PageIndex{2}\)
Could a purely electric field propagate as a wave through a vacuum without a magnetic field? Justify your answer.
No. The changing electric field according to the modified version of Ampère’s law would necessarily induce a changing magnetic field.

An official website of the United States government
Official websites use .gov A .gov website belongs to an official government organization in the United States.
- JetStream home
- Layers of the Atmosphere
- Air Pressure
- The Transfer of Heat Energy
- Energy Balance
- Hydrologic Cycle
- Precipitation
- Layers of the Ocean
- Ocean Circulations
- The Sea Breeze
- The Marine Layer
- Rip Current Safety
- Global Atmospheric Circulations
- The Jet Stream
- Climate vs. Weather
- Climate Zones
- How clouds form
- The Core Four
- The Basic Ten
- Cloud Chart
- Color of Clouds
- Parcel Theory
- Stability-Instability
- Radiosondes
- Skew-T Log-P Diagrams
- Skew-T Plots
- Skew-T Examples
- Pressure vs. Elevation
- Longwaves and Shortwaves
- Basic Wave Patterns
- Common Features
- Thickness Charts
- Weather Models
- Surface Weather Plots
- Norwegian Cyclone Model
- Types of Weather Phenomena
Electromagnetic waves
- The Atmospheric Window
- Weather Satellites
- How radar works
- Volume Coverage Patterns (VCP)
- Radar Images: Reflectivity
- Radar Images: Velocity
- Radar Images: Precipitation
- Inter-Tropical Convergence Zone
- Classification
- NHC Forecasts
- Hazards & Safety
- Damage Potential
- ENSO Pacific Impacts
- ENSO Weather Impacts
- Ingredients for a Thunderstorm
- Life Cycle of a Thunderstorm
- Types of Thunderstorms
- Hazard: Hail
- Hazard: Damaging wind
- Hazard: Tornadoes
- Hazard: Flash Floods
- Staying Ahead of the Storms
- How Lightning is Created
- The Positive and Negative Side of Lightning
- The Sound of Thunder
- Lightning Safety
- Frequently Asked Questions
- Types of Derechos
- Where and When
- Notable Derechos
- Keeping Yourself Safe
- Historical Context
- Causes: Earthquakes
- Causes: Landslides
- Causes: Volcanoes
- Causes: Weather
- Propagation
- Detection, Warning, and Forecasting
- Preparedness and Mitigation: Communities
- Preparedness and Mitigation: Individuals (You!)
- Select NOAA Tsunami Resources
- Weather Forecast Offices
- River Forecast Centers
- Center Weather Service Units
- Regional Offices
- National Centers for Environmental Prediction
- NOAA Weather Radio
- NWS Careers: Educational Requirements
- Weather Glossary
- Weather Acronyms
- Learning Lessons
- Electromagnetic Waves
- Atmospheric Absorption
Electromagnetic waves are a form of radiation that travel though the universe. They are formed when an electric field (Fig. 1 red arrows) couples with a magnetic field (Fig.1 blue arrows).
Both electricity and magnetism can be static (respectively, what holds a balloon to the wall or a refrigerator magnet to metal), but when they change or move together, they make waves. Magnetic and electric fields of an electromagnetic wave are perpendicular to each other and to the direction of the wave.
Unlike sound waves, which must travel through matter by bumping molecules into each other like dominoes (and thus can not travel through a vacuum like space), electromagnetic waves do not need molecules to travel. They can travel through air, solid objects, and even space, making them very useful for a lot of technologies.
When you listen to the radio, connect to a wireless network, or cook dinner in a microwave oven, you are using electromagnetic waves. Radio waves and microwaves are two types of electromagnetic waves. They only differ from each other in wavelength – the distance between one wave crest to the next.
While most of this energy is invisible to us, we can see the range of wavelengths that we call light. This visible part of the electromagnetic spectrum consists of the colors that we see in a rainbow – red, orange, yellow, green, blue, indigo, and violet. Each of these colors also corresponds to a different measurable wavelength of light.
Waves in the electromagnetic spectrum vary in size from very long radio waves that are the length of buildings to very short gamma-rays that are smaller than the nucleus of an atom.
Their size is related to their energy. The smaller the wavelength, the higher the energy. For example, a brick wall blocks the relatively larger and lower-energy wavelengths of visible light but not the smaller, more energetic x-rays. A denser material such as lead, however, can block x-rays.
While it’s commonly said that waves are "blocked" by certain materials, the correct understanding is that wavelengths of energy are absorbed by the material. This understanding is critical to interpreting data from weather satellites because the atmosphere also absorbs some wavelengths while allowing others to pass through.

What is an electromagnetic wave quizlet?
Electromagnetic Waves. a transverse wave that transfers electrical and magnetic energy. Electromagnetic Waves consist of. vibrating electric and magnetic fields that move through space at the speed of light. Electric Field.
What are electromagnetic waves simple definition?
Definition of electromagnetic wave : one of the waves that are propagated by simultaneous periodic variations of electric and magnetic field intensity and that include radio waves, infrared, visible light, ultraviolet, X-rays, and gamma rays.
What is a wave quizlet physics?
A wave is a travelling disturbance that travels through space and matter transferring energy from one place to another. energy transfer. Movement of energy from one place or object to another. crest.
Which is the best definition of electromagnetic energy?
Electromagnetic energy is radiant energy that travels in waves at the speed of light. It can also be described as radiant energy, electromagnetic radiation, electromagnetic waves, light, or the movement of radiation. Electromagnetic radiation can transfer of heat.
What are electromagnetic waves made of quizlet?
An electromagnetic waves is made up of vibrating electric and magnetic fields that move through space or some medium at the speed of light.
What is electromagnetic energy quizlet?
electromagnetic energy is defined as. A form of energy that travels through space as waves. What are radio waves. Electromagnetic waves with the longest wavelengths and lowest frequencies.
Which are electromagnetic waves?
The electromagnetic spectrum is generally divided into seven regions, in order of decreasing wavelength and increasing energy and frequency. The common designations are radio waves, microwaves, infrared (IR), visible light, ultraviolet (UV) light, X-rays and gamma-rays.

What is electromagnetic wave and their examples?
When you listen to the radio, watch TV, or cook dinner in a microwave oven, you are using electromagnetic waves. Radio waves, television waves, and microwaves are all types of electromagnetic waves. They only differ from each other in wavelength. Wavelength is the distance between one wave crest to the next.
What is other term for electromagnetic waves?
Electromagnetic Wave synonyms Radiation (quantized as photons) consisting of. 0. 0. Find another word for electromagnetic wave. In this page you can discover 2 synonyms, antonyms, idiomatic expressions, and related words for electromagnetic wave, like: nonparticulate radiation and electromagnetic radiation.
What causes waves physics quizlet?
~A wave is caused by a disturbance in a solid, liquid, or gas as energy is transmitted through a medium.
What is frequency in physics quizlet?
Frequency. the number of times the motion repeats itself in a time interval of one unit of time is known as the frequency of the wave. ( Hz) Hz.
What causes a wave in science?
Waves are most commonly caused by wind. Wind-driven waves, or surface waves, are created by the friction between wind and surface water. As wind blows across the surface of the ocean or a lake, the continual disturbance creates a wave crest.
What is electromagnetic waves and its properties?
Electromagnetic waves are members of a family of waves with common properties called the electromagnetic spectrum. All electromagnetic waves: are transverse waves; can travel through a vacuum ; travel at exactly the same speed in a vacuum, the speed of light, 300,000,000 m/s.
Why is electromagnetic waves important?
Electromagnetic waves are used to transmit long/short/FM wavelength radio waves, and TV/telephone/wireless signals or energies. They are also responsible for transmitting energy in the form of microwaves, infrared radiation (IR), visible light (VIS), ultraviolet light (UV), X-rays, and gamma rays.
What is the most important electromagnetic wave?
Electromagnetic waves are classified according to their frequency. The different types of waves have different uses and functions in our everyday lives. The most important of these is visible light, which enables us to see.
How are electromagnetic waves different from other waves?
Electromagnetic waves differ from mechanical waves in that they do not require a medium to propagate. This means that electromagnetic waves can travel not only through air and solid materials, but also through the vacuum of space.
What is the source of all electromagnetic waves?
Solution : Electromagnetic waves are generated due to changes of electric and magnetic fields associated with oscillating charges.
What can electromagnetic waves travel through quizlet?
Electromagnetic waves can travel through a vacuum, or empty space, as well as through matter. The transfer of energy by electromagnetic waves traveling through matter or across space is called electromagnetic radiation.
How are the different kinds of electromagnetic radiation described quizlet?
electromagnetic radiation is classified by wavelength into radio wave, microwave, terahertz (or sub-millimeter) radiation, infrared, the visible region that is perceived as light, ultraviolet, X-rays and gamma rays. The behavior of EM radiation depends on its wavelength.
Which type of wave has the longest wavelength?
Radio Waves — The waves in the electromagnetic spectrum that have the longest wavelengths and lowest frequency are called radio waves.
Which of the following describes light as a wave quizlet?
Which of the following describes light as a wave? Light is a transverse wave.
Why is it called electromagnetic waves?
In other words, EM waves are composed of oscillating magnetic and electric fields. Description: Electromagnetic waves are formed when an electric field comes in contact with a magnetic field. They are hence known as ‘electromagnetic’ waves.
How do you identify electromagnetic waves?

How are electromagnetic waves classified?
Generally, electromagnetic radiation is classified by wavelength into radio wave, microwave, infrared, visible light, ultraviolet, X-rays and gamma rays. The behavior of EM radiation depends on its wavelength.
Who produce electromagnetic waves?
Electromagnetic waves are produced whenever electric charges are accelerated. This makes it possible to produce electromagnetic waves by letting an alternating current flow through a wire, an antenna. The frequency of the waves created in this way equals the frequency of the alternating current.
Privacy Overview

IMAGES
VIDEO
COMMENTS
Diffraction. When light waves bounce away from surface which do not absorb them. Reflection of light. Study with Quizlet and memorize flashcards containing terms like Electromagnetic waves can also be called, The transfer of energy by electromagnetic waves., Electromagnetic waves do not need a _________ to travel through. and more.
Electromagnetic Radiation. The transfer of energy by waves traveling through matter or empty space. Electromagnetic Wave. A wave that consists of vibrating electric and magnetic fields. Magnetic Field. Only acts on moving electrons. Electric Field. Acts on all charged particles, moving or not. Transverse Wave.
Study with Quizlet and memorize flashcards containing terms like Electromagnetic Waves, Electromagnetic Radiation, Medium and more. ... All electromagnetic waves travel at the same speed in a vacuum. 300,00km/speed. Earth. At this speed it takes light 8 minutes to travel the 150 million kilometers from the sun to_____.
electromagnetic radiation consists of electromagnetic waves, which are synchronized oscillations of electric and magnetic fields. It includes radio waves, microwaves, infrared, (visible) light, ultraviolet, X-rays, and gamma rays. In a vacuum electromagnetic waves travel at the speed of light, commonly denoted c.
Q-Chat. Study with Quizlet and memorize flashcards containing terms like Energy from the sun reaches Earth by traveling in, A wave that can travel through empty space and matter, energy carried by an electromagnetic wave and more.
Which lists the waves in order of frequency, from highest to lowest? X-rays, gamma rays, ultraviolet. radio waves, visible light, X-rays. ultraviolet, microwaves, gamma rays. visible light, infrared, microwaves. visible light, infrared, microwaves. Violet light has a frequency of 7.26 × 1014 Hz and travels at a speed of 3.00 × 108 m/s.
Electromagnetic radiation is one of the many ways that energy travels through space. The heat from a burning fire, the light from the sun, the X-rays used by your doctor, as well as the energy used to cook food in a microwave are all forms of electromagnetic radiation. While these forms of energy might seem quite different from one another ...
And the speed at which these waves travel is the speed of light, c, and by c I mean three times 10 to the eight meters per second, because light is just and Electromagnetic wave, light is a special example, one particular example of Electromagnetic waves, but it is only one example, these waves can have any wavelength.
Introduction. Electromagnetic waves have two components: an oscillating electric field and a perpendicular, comoving magnetic field which oscillates at the same frequency, but with a phase shifted by 90°. They describe the movement of a packet of energy between two points. In the discussion of EM waves, we are normally concerned with its ...
Electromagnetic waves. Electromagnetic radiation, is a form of energy emitted by moving charged particles. As it travels through space it behaves like a wave, and has an oscillating electric field component and an oscillating magnetic field. These waves oscillate perpendicularly to and in phase with one another.
Radio waves are a type of electromagnetic (EM) radiation with wavelengths in the electromagnetic spectrum longer than infrared light. They have have frequencies from 300 GHz to as low as 3 kHz, and corresponding wavelengths from 1 millimeter to 100 kilometers. Like all other electromagnetic waves, radio waves travel at the speed of light.
What is Electromagnetic energy? Electromagnetic energy travels in waves and spans a broad spectrum from very long radio waves to very short gamma rays. The human eye can only detect only a small portion of this spectrum called visible light. A radio detects a different portion of the spectrum, and an x-ray machine uses yet another portion.
The photons that make up radio waves travel at the same speed as the photons that make up visible light. [A] True. [B] False. [C] We don't know. The electromagnetic spectrum can be expressed in terms of energy, wavelength, or frequency. [A] True. [B] False. Low energy photons are waves.
An electromagnetic wave can also be described in terms of its energy—in units of measure called electron volts (eV). An electron volt is the amount of kinetic energy needed to move an electron through one volt potential. Moving along the spectrum from long to short wavelengths, energy increases as the wavelength shortens.
Magnetic fields are generated by moving charges or by changing electric fields. This fourth of Maxwell's equations, Equation 16.2.17, encompasses Ampère's law and adds another source of magnetic fields, namely changing electric fields. Maxwell's equations and the Lorentz force law together encompass all the laws of electricity and magnetism.
electromagnetic spectrum, the entire distribution of electromagnetic radiation according to frequency or wavelength.Although all electromagnetic waves travel at the speed of light in a vacuum, they do so at a wide range of frequencies, wavelengths, and photon energies. The electromagnetic spectrum comprises the span of all electromagnetic radiation and consists of many subranges, commonly ...
Electromagnetic radiation is a form of energy that can travel through space and matter. It includes visible light, radio waves, microwaves, infrared, ultraviolet, X-rays and gamma rays. Learn more about the properties, sources, effects and applications of electromagnetic radiation from this Wikipedia article.
An electromagnetic wave continues to go straight and spread out until it collides with some form of matter, at which point the direction of travel will change. Slide 11 / 147 Electromagnetic Wave Characteristics Electromagnetic waves have the same wave characteristics we studied last unit: wavelength (λ) measured in meters frequency (f ...
Radio waves have the longest wavelengths in the electromagnetic spectrum. They range from the length of a football to larger than our planet. Heinrich Hertz proved the existence of radio waves in the late 1880s. He used a spark gap attached to an induction coil and a separate spark gap on a receiving antenna. When waves created by the sparks of ...
Electromagnetic waves. Download Image. Electromagnetic waves are a form of radiation that travel though the universe. They are formed when an electric field (Fig. 1 red arrows) couples with a magnetic field (Fig.1 blue arrows). Both electricity and magnetism can be static (respectively, what holds a balloon to the wall or a refrigerator magnet ...
electromagnetic radiation, in classical physics, the flow of energy at the universal speed of light through free space or through a material medium in the form of the electric and magnetic fields that make up electromagnetic waves such as radio waves, visible light, and gamma rays.In such a wave, time-varying electric and magnetic fields are mutually linked with each other at right angles and ...
Electromagnetic waves are members of a family of waves with common properties called the electromagnetic spectrum. All electromagnetic waves: are transverse waves; can travel through a vacuum ; travel at exactly the same speed in a vacuum, the speed of light, 300,000,000 m/s.